The Beauty of Physics
Join my journey from the mid-galactic black-hole to the keratin in your hair, and discover the beauty of physics.
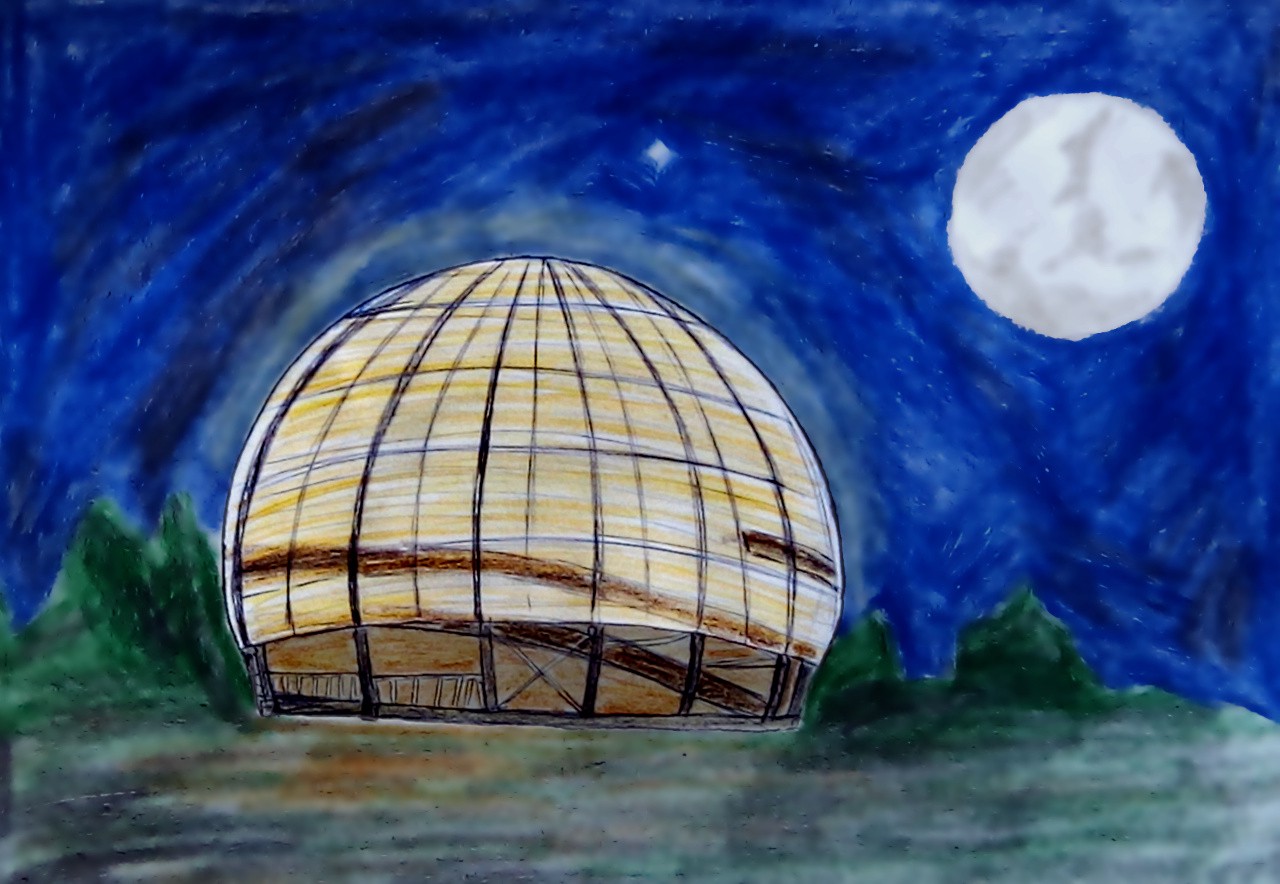
Join my journey from the mid-galactic black-hole to the keratin in your hair, and discover the beauty of physics.
The evening before my departure to Geneva, Switzerland, I was looking up in the sky at the moon, which was a waxing crescent that evening. Tomorrow was a school trip to CERN, the European Organization for Nuclear Research, and that set me thinking.
I know that we can only see one half of the moon because that’s the only side that’s illuminated but never thought about the physics aspect of it: we can see that part since the rays of light are reflected towards our eyes, which isn’t happening on the dark side. That’s when I realised how beautiful physics can be.
We arrived at CERN in the late morning of Wednesday. The look reminded me of an industrial park, with its chain-link fence and large, glass-windowed buildings. A voluntary guide led us to a classroom, where we all sat very close to each other, small wooden planks in front of us serving as writing-tables.
The guide showed us a video which gave more information about what they do at CERN and how they do it, by example: at the LHCb they try to figure out why there was a matter-surplus at the Big Bang. This surplus is the reason our universe exists today. It was a good video but unfortunately it crashed halfway. Not to be perturbed, guide went on to tell us about the other things that would’ve been shown — but he got me really excited when he started talking about antiparticles.
The antiparticle of the proton, he explained, is the “antiproton”, and the antiparticle of the electron is the antielectron or “positron”. Together, they can form an antihydrogen atom, just as ordinary protons and electrons form hydrogen. What’s more, this “antihydrogen” has exactly the same properties, except charge which is opposite, as a hydrogen atom.
When particles and antiparticles touch, they annihilate each other — both vanishing like a ball of water filling a bubble in the ocean, but releasing photons of energy in the process.
At CERN, one of the things they try to figure out is why our universe consists almost entirely of matter, rather than being a mixture of matter and antimatter.
After our guide’s introductory speech, we went to Compact Muon Solenoid, which is the other end of the more well-known Large Hadron Collider.
The Large Hadron Collider, or LHC, is a particle collider with a 27-kilometre-long circuit. Protons, lead ions, and other such “hadrons” are sent round this circuit at incredibly high speeds — sometimes spinning round at 11,000 loops per second. Lead ions are used during a “heavy ion” run where lead ions are collided.
Giant superconducting magnets guide these particles into two beams, which cross over at 4 points. These points, LHCb, ATLAS, CMS and ALICE, are where the particles smash head-on into each other, sometimes even recreating conditions similar to billionths of seconds after the Big Bang.
By smashing these particles together and seeing what comes out, scientists hope to answer some of the burning questions facing physics today. These include “dark matter”, the hypothetical stuff that’s thought to account for 25% of the matter in the universe. Scientists suspect it exists because the gravity of the Universe behaves as if there was.
Then there’s supersymmetry, the theory that every known particle has a heavier counterpart. These could explain dark-matter, and the LHC is on the lookout for them.
The LHC also investigates the various “string theories”, the different multidimensional models of how the Universe may be put together. What the LHC does is to collide particles, then add up the resultant energy. If it doesn’t add up, there’s a chance that maybe the missing energy ended up in another dimension.
These are some of the big questions currently being faced by physics — which also means being faced by everyone in the world. Interestingly, the LHC attempts to answer them, not by looking everywhere at once, but by concentrating on even tinier and tinier areas.
In some way you can say that everything came into existence thanks to physics and is kept this way thanks to it.
The moon, for example, turns around the earth, which turns around the sun and the sun turns around a black hole in the middle of our galaxy. This is all caused by gravitational pull which simply is a force that massive objects practice on each other and causes them to get attracted.
But that’s not all. The size of the pull follows a precise mathematical pattern, given by Newton’s law of universal gravitation:
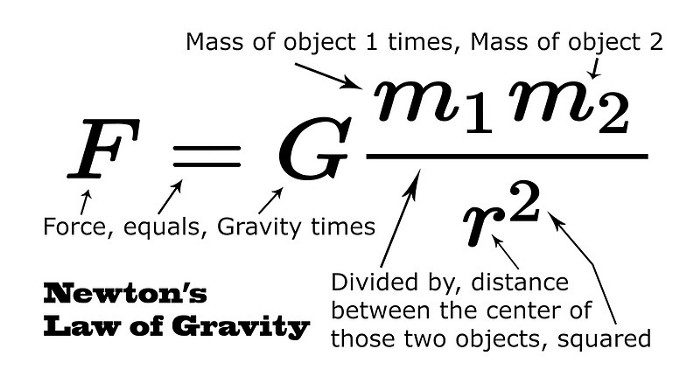
That might look complicated at first, but it’s quite simple if you think about it. If m₁ and m₂ are the masses of two objects in space, you get the force by multiplying the masses, and dividing by the distance between them. (The ‘G’ is just a constant to scale down the numbers: the way you multiply centimetres by 10 to get millimetres).
The heavier the masses, the larger the gravitational pull; the shorter the distance, the stronger the pull will get. That’s all there is to it.
And yet, this rule somehow holds the moon, the earth, the sun, and indeed the whole Universe under its command.
The Compact Muon Solenoid, other side of the LHC, is located in France: a twenty-minute drive for us by commissioned CERN bus. At first it looked like a typical business building if slightly shorter and broader than the previous one, but then a guide took us to the back to talk about safety measures for going underground.
Like the LHC, the Compact Muon Solenoid is located under the ground. Particles are sent in from one side of a kilometres-long passageway (CERN’s main campus), to accelerate in both directions and collide where detectors are located. One of those detectors is the Compact Muon Solenoid, or CMS, located at the other end of the LHC.
The guides gave us orange helmets with the CMS logo on them, just like the ones they themselves were wearing. However they also had on yellow fluo jackets, while we stayed in our ordinary clothes.
We took a lift down to the highest floor underground — an eighty-metre trip in a smooth metal box, with a wall-mounted TV playing safety instructions all the way.
Since we were only going one level down the journey was over in half a minute; however my ears popped, like they do on an airplane, which is something I hate a lot.
We arrived in a cavern made of concrete and equipped with lots of pipes running above us. The guide explained to us how the CMS works, then took us to a second cavern without corridors: one that was only accessible because the LHC is currently in shutdown.
The LHC, I learnt, is undergoing a two-year upgrade process: to increase the amount of particles in the beam, as well as to repair the CMS. That was lucky for me, because it meant I had a chance to see the detector. Along with ATLAS (A Toroidal LHC ApparatuS) it discovered the Higgs boson (an elementary particle in the Standard Model of particle physics) in 2012.
When we came into the cavern I was amazed that such a huge apparatus is used to detect very small particles.
It looked like an enormous circular metal construction, packed with all kinds of electronics and wires in a concentric formation. Weighing 14.000 tonnes — nearly twice the weight of the Eiffel Tower — its smallest ring on the inside was itself the size of an aeroplane. It consists of two parts set on red platforms equipped with wheels so they can be rolled apart. It was an amazing experience and made me more amazed by physics and its beauty. I hope to go back to CERN one day and maybe even work there. The chance may be small, but never say never.
I have mostly talked about how physics are important and beautiful in a large scale but the same counts for the small.
Think about your hair. It’s made of keratin compounds which are made up of different subunits, amino acids. These amino acids have atoms like carbon, nitrogen, oxygen, hydrogen and sometimes sulphur. Each one of these atoms are a collection of protons, neutrons and electrons. These are kept together thanks to their charges; assembled once long ago in the heart of a star.
This is a part of physics too, more specifically electrostatics.
Science is all about learning how the world works. You look at something, you take it apart, you investigate it to figure out the laws that make it run. And the science that takes this investigation to the deepest level, on the smallest scale as well as the largest, is physics. It is through physics that you learn why atoms bond they way chemistry tells us; how light travels through the biologist’s eye is explained by physics again.
One can find many interesting patterns at levels high and low, but physics looks right at the fundamental laws of the Universe: the deepest level of all.
Thanks to Lauren Yeomans, CERN physicist, for help reviewing this piece.
Want to write with us? To diversify our content, we’re looking out for new authors to write at Snipette. That means you! Aspiring writers: we’ll help you shape your piece. Established writers: Click here to get started.