Superconductors
The search for room-temperature zero-resistance materials.
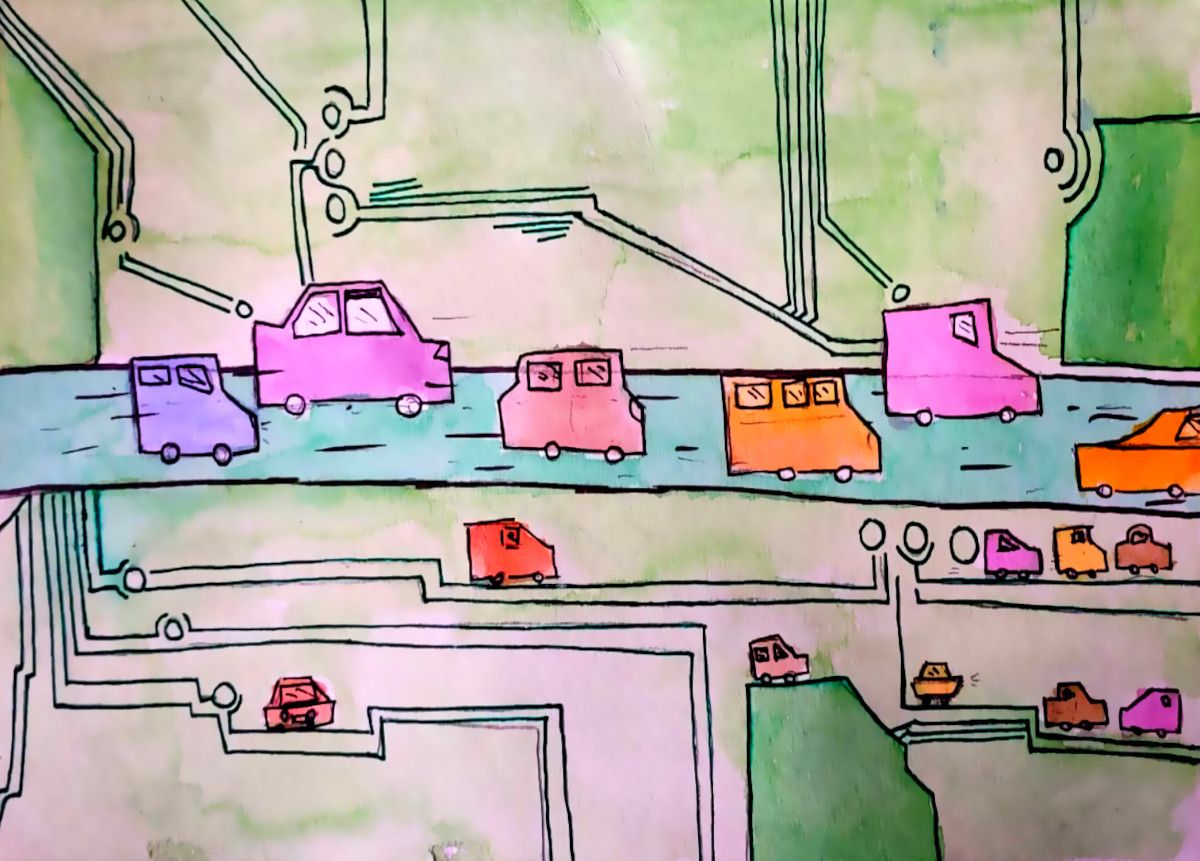
The search for room-temperature zero-resistance materials.
The loud, angry honks of cars punctuate the early morning silence. One follows another, a cacophony of sound that is enough to drive anyone crazy! You navigate your car as carefully as you can, cruising past some, and slowing to follow others. Eventually you are forced to come to a complete stop. It’s a massive traffic jam.
Electricity flows much like a car on a busy road, though only through materials that allow currents to pass through it. These materials are known as conductors. Conversely, there is another set of materials, which, like the jam, does not allow electricity to flow through it. Those are called insulators.
Conductors and insulators — two terms we use in our everyday lives. We see the former daily, in metal wires; and the latter in items like rubber, or plastic. But here’s something interesting — even though conductors allow current to flow through them, they’re imperfect. When current flows through a conductor, the moving electrons bump into atoms and lose energy–kind of like how cars are forced to constantly move and stop on roads. This loss of energy is called resistance, measured in ohms.
But what if we were driving on a deserted highway instead, with nothing to stop us cruising away into the sunset?
I am talking, as you may have guessed, about superconductors.
Superconductors are materials in which electric currents can persist indefinitely — an eternal road, so to speak. In the last century, the discovery of this type of material has fascinated scientists primarily because of two important reasons.
Think of a river. As it flows on to the ocean, it usually gets faster and faster , picking up space and developing into an enormous beast — but that’s only because more water flows in and joins it along the way. Left to run alone, as happens in an artificial canal, this river would lose water over time to soaking and evaporation, until it gets reduced to a tiny trickle.
Electricity faces a similar resistance, which is why you usually don’t end up with the same amount you started with. But certain materials, when cooled to a low temperature, lose all electrical resistance, much like a pipe insulates water from the elements. These materials become perfect conductors, known as superconductors.
That’s only one of the reasons people like superconductors. The other reason is that they allow trains to float.
When you bring a magnet close to a conductor, the electrons in the conductor feel this changing magnetic field, and start moving in a circle called an eddy current. This eddy current counteracts the magnetic field by generating its own magnetic field.
In a normal conductor, the eddy current dies away quickly because of electrical resistance. However, in a superconductor, there’s no resistance to speak of, allowing the eddy currents circulate practically forever. This moving current generates a magnetic field that cancels out the original magnetic field, opposing it and causing the superconductor to magically levitate above.
This property, the Meissner effect, is what is used for technological innovations like the Maglev, a train that runs by hovering over the tracks instead of running on them, eliminating friction and allowing it to travel at fantastic speeds.
In the last three decades, researchers discovered that even some insulators could be cooled down to become superconductors. As these materials superconducted at higher temperatures than before they are called ‘high-temperature superconductors’, even though these “high” values are still way below room temperature.
These materials were revolutionary because you could now study them with just liquid nitrogen at just 77 Kelvin (-196 C), which is way cheaper than the previously used liquid helium which needed to be cooled down to 4 Kelvin (-269 C)
For reference, the room temperature is about 295 Kelvin (21 C), no minus sign included.
Based on copper, iron, or pure carbon, high-temperature superconductors made it easier for researchers to work, but they needed to be put under extreme pressures to superconduct. I’m talking levels that would be normal for a typical outdoor environment…in Jupiter. On Earth, we recreate it with ‘diamond anvil cells’, which press the material hard between two diamonds.
Trains aside, another way to use a superconductor is to circulate current round and round, building up a frenzily high magnetic field. This principle is used in MRI machines, that read distortions in this magnetic field to generate an image of your brain or body.
On a larger scale, scientists are using it to produce fields in tokamak reactors, in their quest to develop controlled nuclear fusion.
Most materials become superconductors only when under a specific level of pressure, and below a specific temperature that’s usually very low. But what if we could develop superconductors that worked at room temperature, and normal atmospheric pressure? These would allow us to set up superconductors out in the open, giving rise to many more possibilities.
Starting with the obvious one: transporting electricity without losing some of it on the way.
In recent years, another set of materials called hydrides were discovered to be specifically good at becoming superconductors.
In 2020, a room-temperature superconductor was claimed to be discovered, which superconducts under a huge amount of pressure. However, this study was retracted because other scientists had trouble recreating their results. Retracting a paper in science is a huge deal because it breaks others’ trust in the group.
But now, the same team report superconductivity in another material — nitrogen-doped lutetium hydride with a maximum Tc of 294 K. This time, however, other scientists are confident that the analysis was thorough. The team observed zero resistance in the material and showed that the material exhibited the Meissner effect: it holds train-levitating potential.
To measure the Meissner effect, they used a vibrating-sample magnetometer or VSM. Using this method, a sample is placed in a constant magnetic field and moved up and down. Depending on the magnetising properties of the sample, this vibrating magnetic field induces a proportional electric current in the pickup coils of the VSM, which allows us to measure the magnetic properties of the sample.
The material in question is bluish at high temperatures, and as the temperature was lowered, it became pink and superconductive after which it became red and non-superconductive again. It only becomes superconductive at room temperature at a pressure of about 10,000 atmospheric pressures. This is admittedly higher than what we expect from a regular room, but dramatically lower than previous experiments.
The way the atoms are arranged in this material is at the heart of why it superconducts. This is why scientists are interested in studying its composition, using different analyses: X-ray diffraction (XRD), Energy Dispersive X-ray spectroscopy (EDX), and Raman spectroscopy.
XRD and EDX use X-rays to study the crystal structure of a material. X-rays are sent in all directions onto a crystalline material. By studying how the X-rays are scattered, information about the elements within, the crystal structure, and lattice spacing can be obtained.
Raman spectroscopy uses laser light to study the material. When a laser is directed onto a material, the scattered light lets us study the vibrational modes of the chemical bonds within the material — which gives information about the different kinds of bonds in a material.
These analyses showed the presence of two distinct hydride compounds — each with a face-centred cubic (fcc) metal sub-lattice but with varying contents of hydrogen and nitrogen.
However, more experiments are needed to determine the exact crystal structure of the material.
Exciting as it sounds, this research is unlikely to change our lives just yet. many things stand in it’s way. It requires extremely expensive laboratory equipment and very high pressure. The latter is especially harder to recreate here too. Moreover, this study cannot be reproduced by other research groups because some key details of how to make the material are still missing. The research was funded by a company, and they continue to own intellectual rights, making those details inaccessible to the public.
Maybe we’ll figure it all out someday — but until then, traffic jams and normal trains are what we have.