Field Study
Using flint to unearth the history of our planet’s magnetic field.
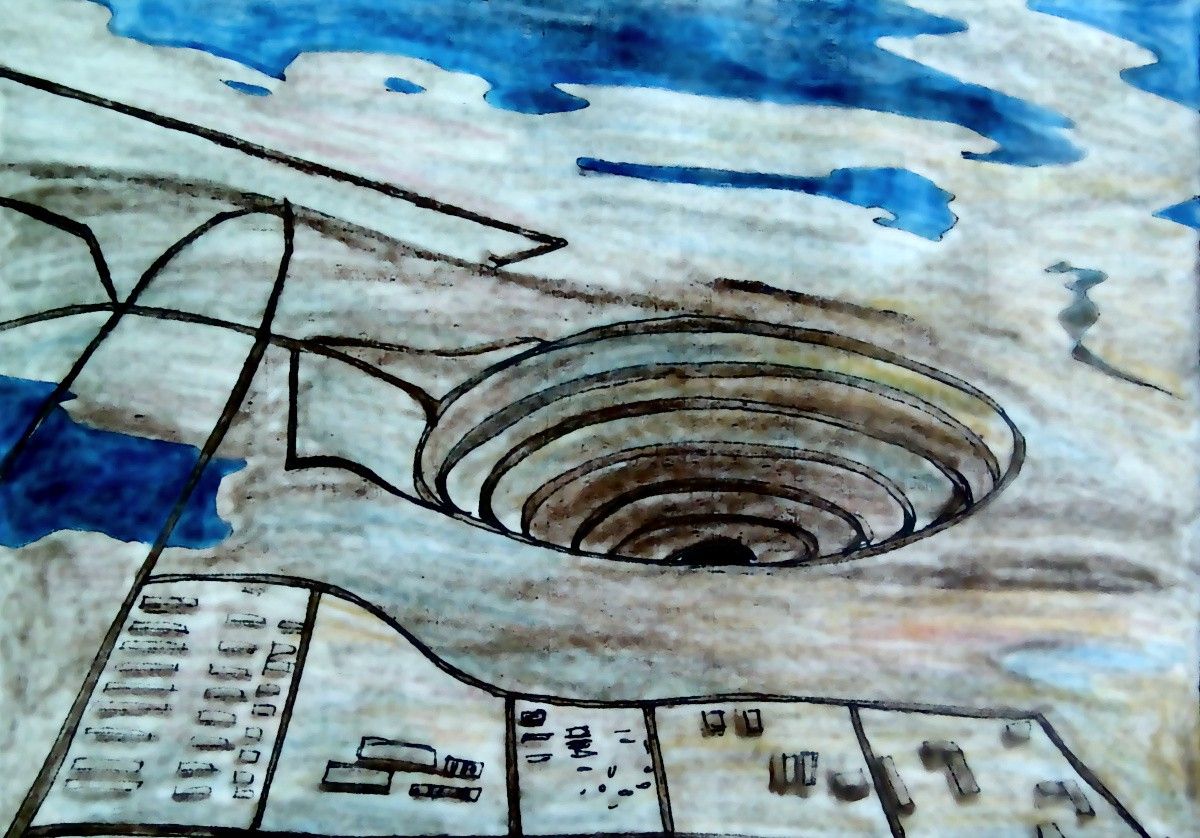
Using flint to unearth the history of our planet’s magnetic field.
Humanity has been to the Moon and back, but we haven’t yet travelled to the centre of our own planet. And that’s not without reason: drilling into the depths of the Earth is an undertaking comparable to rocket science — or harder.
Before the Space Race, where two enemy nations competed to land their citizen on the Moon before the other, there was an equally frantic competition to drill into the depths of the Earth. This environment is evidently more hostile than outer space, because these “expeditions” were completely unmanned. They involved boring a hole into the ground, much like a borewell but deeper, and then sending in sensors and probes to find what they could.
The deepest such project was the Kola borehole, drilled over the course of twenty-four years in Russia’s Kola peninsula.
The Earth can be imagined as a cake with distinct layers. Below the icing of life and biodiversity lies the hard, crunchy crust on which we stand — which, while thin on the scale of things, is still so thick that even the Kola borehole never went right through it. The deeper you go, the hotter it gets: after the crust is a mantle of solid rock on which our continents stand, followed by a creamy molten nickel-iron liquid. And finally, right in the centre, is a core of solid iron.
Though this cake may send you stomping off to the store to ask for a refund, it does a very good job at protecting us against an invisible enemy.
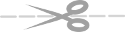
When the Moon-bound Apollo astronauts left the Earth behind and adapted their eyes to the darkness, they began to see sudden coloured flashes of light. These bright flashes became increasingly common — sometimes as often as two per minute. And the most surprising part? They were visible to the astronauts even when their eyes were closed.
This was likely caused by Cerenkov radiation: light produced when highly charged particles pass rapidly through a medium, in this case right inside the astronauts’ eyes. As for the particles, they were solar particles, part of a steady stream that forever emanates from the Sun. If that sounds dangerous, it is — but only in high doses.
Because the astronauts were only out in space for a week or two, they survived solar radiation without much damage — although they did end up with a greater chance of developing cataracts in their eyes. Solar particles are a big problem for longer missions though, and one of the reasons humans haven’t yet reached Mars. At those levels of exposure, astronauts would have a significantly higher chance of developing cancer and other complications.
But if a six-month trip to Mars can be so harmful, what about us here on Earth, being bathed is solar particles every day? Luckily that doesn’t happen, because solar particles are magnetic — and the Earth’s layers have the ideal defence.
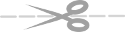
The Kola borehole ended at the depth of 12,200 metres, but it barely scratched the surface of the planet. Even at that depth, temperatures reached 180 °C, hot enough to bake a cake and melt any machinery that tried to drill further. But if you wanted to descend to the true centre of the Earth, you’d have to descend well more than five hundred times that distance.
It is at these depths that hold key to the creation of the Earth’s magnetic field.
This magnetic field has allowed sailors to navigate around the world using their compass. It is responsible for the beautiful auroras observed near the North Pole, and, last but not the least, it protects us from the solar particles being thwarted towards us.
In the last century, though, we learnt that this field is actually constantly shifting. Sometimes, it can flip altogether — North and South will switch places, and all our compasses will go in reverse.
This changing field is a problem because, without it, the solar particles will destroy our planet.
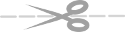
William Gilbert was an English physician, physicist, and natural philosopher. He is best known for a work published in 1600, where he proposed that our planet itself is a large magnet and studying the magnetic field gives us an unprecedented insight into its heart.
To understand how the Earth might generate a magnetic field, the main principle to remember is Faraday’s Law or the dynamo theory which states that a moving electric field generates and magnetic field and vice versa. That is, after all, how power plants generate electricity: whether through coal, water, wind, or nuclear power, their main aim is to keep powerful magnets continuously moving. When the magnetic field moves, the movement creates an electric field which is picked up by wires and hopefully delivered to your home.
Faraday’s Law is also how electric motors work. It’s basically the same principle, but in reverse: current travelling through a wire generates a magnetic field, which pushes or pulls on the magnets of a motor and causes them to spin.
And how did the Earth itself manage to become magnetic? The answer, again, lies in spinning.
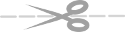
The most prominent explanation for how Earth generates its magnetic field is the geodynamo theory. According to this theory, the system of electric currents in the Earth’s outer core generate the magnetic field.
The process that you observe when making tea where hot water rises and cooler water sinks. The liquid iron-nickel in the Earth’s outer core . All this moving around of the charged material creates electric currents which generates magnetic fields. Because the earth is rotating, the small magnetic fields generated by these currents align to create the big dipolar magnetic field — essentially having a north and south pole — that we observe on the surface.
In the last century, we realised that this magnetic field is also constantly changing. It might seem impossible to study the changes to the magnetic field of the Earth that have happened say 1000 years ago. But astonishingly, this can be achieved by studying volcanic rocks.
To understand how that works, recall that a magnetic material can exist in two phases: magnetised and non-magnetised, depending on the temperature.
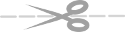
Temperature is nothing but how much atoms move inside a inside a substance. In magnetic materials, each atom has a magnetic moment. At low temperatures, below a certain “Curie temperature”, the atoms don’t move much and all the magnetic moments are aligned; this results in a magnetised material like ferromagnets. At a high temperature — above a certain “Curie temperature” — however, if there is no external magnetic field, due to all the jiggling of the atoms around, all the magnetic moments become misaligned and the material becomes demagnetised, that is, they become paramagnetic. If there is an external magnetic field however, the magnetic moments will align in the direction of this field.
Thus when hot molten lava from a volcano starts cooling, its rocks become magnetised in the direction of the local geomagnetic field, thereby fossilising the direction of the earth’s field at the time of formation of the rock. It was by studying these paleomagnetic samples that led to the conclusion that the poles of Earth’s field have reversed several times.
This method of study need not be limited to rocks but can also be applied to ceramics and pottery. In all these methods, the materials are heated to a high temperature before being cooled rapidly and this allows them to contain an imprint of the magnetic field at that time. In this new study, the researchers extended this method to successfully apply it to flint as well.
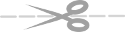
Before the space race, primitive civilisations competed to make better tools. This race was won by inhabitants of Neolithic Western Europe who pioneered the art of making tools from flint.
Imagine you are a primitive human making a new tool. You find that flint, if struck with a harder rock, splinters and flakes away, creating sharp edges and points. This is immensely useful to you because your community has just begun practicing agriculture and you need cutting tools.
Striking flint can be a frustrating process. Sometimes the flint does not flake away, but instead crumbles and cracks. In order to prevent this you treat the flint by heating it for a day and then letting it cool slowly. Now you can easily work the flint and make any manner of tools you want, including weapons!
Little do you know it, but your heat-treatment is also setting clues for your far-future descendants — descendants who go by the names of Anita Di Charia, Lisa Tauxe, Thomas E. Levy, Mohammad Najjar, Fabio Florindo, and Erez Ben-Yosef. These people will go on to author a paper entitled The strength of the Earth’s magnetic field from Pre-Pottery to Pottery Neolithic, Jordan, which will measure shards of pottery and flint tools to read the history of our planet’s magnetic field.
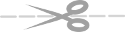
Making magnets is actually very simple. Take a piece of metal, repeatedly run a magnet along it in a consistent direction, and it will eventually turn magnetic too. Or, if you want to get more fancy, run an electric field around your metal, and, because of the Faradaean connection between magnetic and electric fields, you will see that turn magnetic too.
Of course, magnetising a material depends on how agreeable it is to being magnetised. The technical term for this is “susceptibility”, and the way to test it usually involves pushing a material towards a magnet to see how much the material is pulled in or pushed away. The stronger the push, the easier it will be to magnetise the material! Most metals are very susceptible; cakes and bakes not so much.
Just because something is susceptible to being made magnetic doesn’t mean it has already been magnetised. Magnetisation is studied by moving the material inside an electric coil that is not carrying any current. Movement of the magnetic field then generates a current inside the coil which can be measured to find out how magnetic the material is.
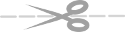
To study how much the magnetic field of the Earth was at the time of formation of their flint and pottery samples, your six descendant researchers have now performed calculations on their susceptibility and magnetism — that is, how susceptible they were to becoming magnetic, and how magnetic they’d actually ended up.
If this ratio of the magnetisation to the susceptibility was very low, the samples were not heated to high enough temperatures to become magnetised well enough. On the other hand, if it was very high, their magnetisations could have been corrupted by lightning or other magnetic fields which make them unsuitable for the study. Only if they matched could the samples be deemed perfect!
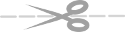
Once they’d narrowed down their samples, the researchers performed something called “Thellier experiments” using the “IZZI protocol”.
Essentially, they heated their samples in a magnetic-field-free environment, and in several steps, to a high temperature. Each time, they measured the magnetisation level at that temperature. They then cooled the samples down in a known magnetic field using the same temperature steps, measuring all the way down as well.
Taking the ratio of these magnetisations, at a given temperature but with and without a known magnetic field, they could compute how much those samples had been magnetised. Taking samples from several time periods, they could see how the magnetism level had changed over time. And there lay the answer to our original question.
When was the last time you rubbed a pot up and down to make it magnetic? Or a knife or blade, for that matter? Probably never. Artefacts like pottery shards and bits of flint were never intentionally magnetised. They picked up their magnetism from that of the magnetic field all around them: the one that emanates from the Earth itself.
We could have made the same measurements with natural geological materials, but human-made specimens can be dated more accurately. This give us a more fine-grained and precise measurement: we can say “the magnetic field was like this 300 years ago” rather than “give or take a few million”.
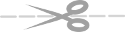
The Earth’s magnetic field is decreasing, and has been doing so for the past 200 years. That means less protection from solar particles. The good news? It’s happened before, and we’ve survived.
Looking at the sample measurements, researchers found that the Earth’s electric field has become very weak in earlier times as well, but after that it strengthened quickly. While we don’t know exactly what mechanisms caused the field to change, at least we know something of what to expect this time.
A look into the Kola borehole, or any other hole that scratches the surface of this planet, is also a look back in time. As you descend, you are presented with a view of generations past: the planet turned edge-on into a vertical scroll-through of aeons past. If you look deep enough, you realise that humans are an exploratory species at heart. We’ve spread across the globe, developing tools along the way, and some of us are now turning our sights skyward. In the process, we encounter new dangers whose history is, ironically, embedded deep under our feet by our own ancestors.
As we work to master the present, what hidden clues are we leaving our future descendants? Only time will tell.