All the Light
Nature has many ways of producing colour. Are some of them coming soon to a printer near you?
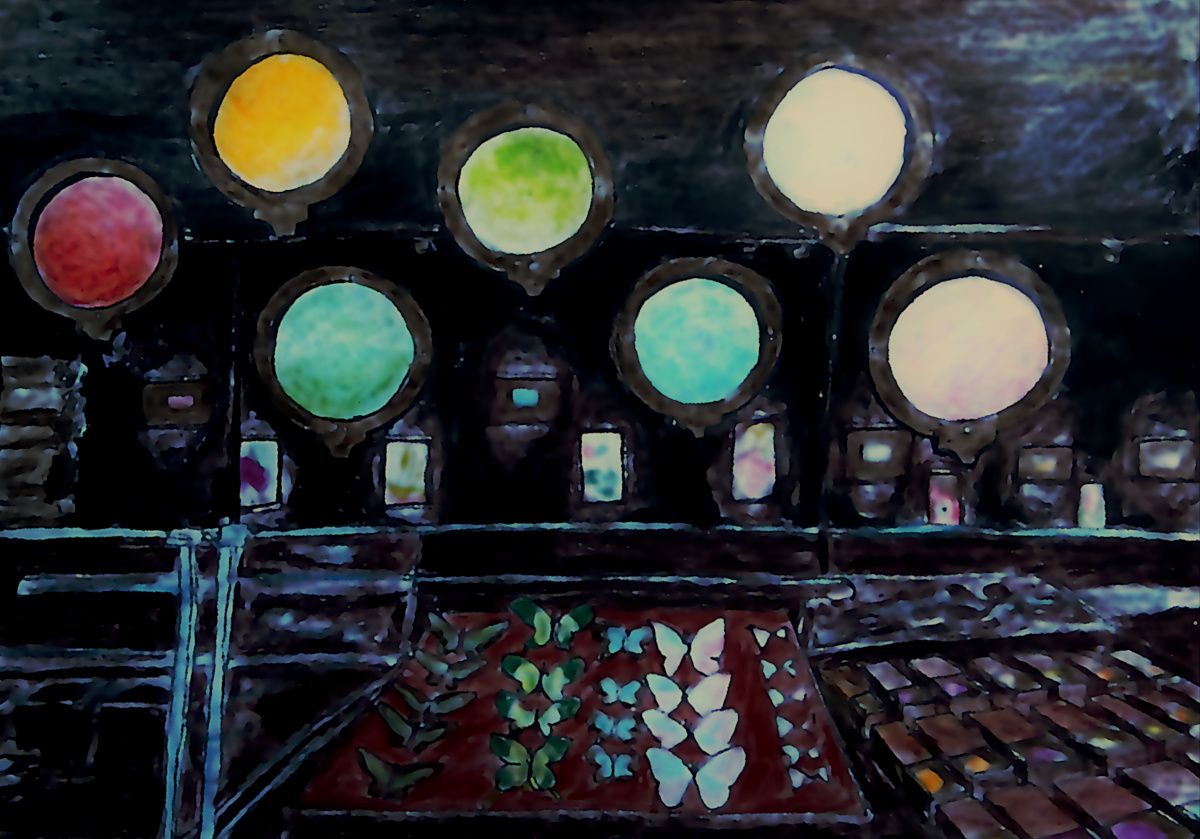
Nature has many ways of producing colour. Are some of them coming soon to a printer near you?
The Royal Botanical Gardens, Kew, is one of the most important botanical institutions in the UK. Founded in 1840 from the exotic garden at Kew Park, it is now home to the world’s largest collection of plants and fungi. Collecting plants isn’t just an end in itself, though: they want to create a world where nature is protected and valued as it should be.
That’s why they make an effort to showcase fancy technology: technology that humans are only starting to work with, but which nature has already mastered.
It was here that I came across a grand exhibition of artwork inspired by the technology behind butterfly wings, soap bubbles, and peacock feathers. Instead of ordinary pigmented paints that could fade over time, these works of art use what is known as “structural colour”. That’s what makes them brighter, longer-lasting, and more scintillating than paintings have even been before in the history of human art.
What is colour? This seemingly simple and familiar phenomenon is more complex than meets the eye.
Colour, to put it technically, is the sensation we perceive when light of a particular wavelength hits our retina. In the physical sense, the wavelength of light is the quantitative property that is related to colour. At low wavelengths, we see shades of blue whereas at high wavelengths, we see shades of red. Of course, if the wavelengths are too low or too high then our eyes can’t sense them and we don’t see them at all — but phones and TVs sometimes do.
It was Sir Isaac Newton’s famous experiment with the prism that set the foundation of the physical nature of colour. The prism split the white light into its constituent parts, revealing all the colours of the rainbow — and Newton realised that it was all those colours, mixed together, that went to form white.
But while seeing colours is easy, producing them isn’t. That’s perhaps why nature has come up with no less than four ways of going about it.
You walk along a large room filled with giant fermentation vats. Inside each is a mixture of wheat bran, sake wine, lime, hardwood ash, and dried leaves from the indigo tree. There it lies, brewing, for three and a half months, until it is ready to use.
Indigo dye used to be a premium product in Europe, a sign of wealth and nobility. It goes to show how important and hard to make a simple colour can be. Ironically, its spread in Japan was as a poor man’s colour: during the Edo period, brighter colours were reserved for richer people, and indigo, with its dozens of possible shades, became the most popular alternative.
Indigo has insect-repellent and anti-bacterial properties, but the main reason for its popularity was that its dye was “fast”: it would wash away with water or fade out over time. Today, synthetically produced dyes play the same role as indigo, but they all work using the same fundamental method of producing colour: pigmentation.
Pigments are materials that absorb most of the light striking them and reflecting back the rest. Leaves absorb everything except green light, which is why you only see the reflected light: green. If you use a green sketch pen over a red sketch pen, the result will be black because, between the two sketch pen lines, all the colours will be absorbed.
Throughout the course of history, artists have been mixing new pigments to produce and display hitherto unused colours. That’s what makes the seemingly frivolous painting IKB 79, so subtle. Composed by artist Yves Klein, it consists of merely a plain blue rectangle — but that blue is a blue that has never been painted with before. More recent is the all-absorbing Vantablack, a black so black that it makes surfaces appear completely flat.
The invention of the printing press suddenly made hitherto obscure and hard-to-find books accessible to all. For a long time, humanity has tried to do the same for visuals and artwork; to produce desired colours in specific forms onto paper.
The latest technology that democratises the production of different colourful shapes and forms is, of course, the printer.
Consider inkjet printers, one of the most common kind in use today. The typical inkjet works by spraying ink onto paper. They usually have only four colours in them: cyan, magenta, yellow, and black. But these colours can be combined in different ways to produce different colours: cyan and yellow, for example, combine to form green. And you can of course increase the proportion of cyan to yellow to get blue-green or yellowish-green or any other kind of green you want.
Sprayed onto small pixels on paper in myriad ways, these colours form all the others we see on printed paper. In other words, by using subpixels with these four colours, pixels of any colour can be generated covering the whole colour gamut. What comes out of printers, though, is essentially pigments. In that sense, it’s using much the same technology as paintings like the IKB 79.
But that’s isn’t the only way to make colours.
The real IKB 79 doesn’t produce any “colour” of its own: it’s just reflecting some specific bits of the light that falls on it. If you shine only red and green light, it’ll end up looking black because it’ll have no blue to reflect back.
On your phone screen, though, you can see a picture of the IKB 79 no matter what light is shining around, because the screen produces light of its own. And so, incidentally, do parts of jellyfish.
When light hits some materials, the electrons in it get temporarily excited to a higher state before jumping back to a lower state. In this process, they release some heat and some light, the latter being what we see as a fluorescent colour. Some sea creatures, most notably the crystal jellyfish Aequorea victoria, use this feature to make themselves glow in the dark using the smallest of light sources.
Scientists have managed to figure out exactly which gene makes the green fluorescent protein, and transfer it to other places…such as neurons in the brain. Its discovery has been something of a boon in neuroscience research, and is now widely used to produce the beautiful images of neuronal activity. By studying the changes in the amount of fluorescence emitted, one can easily study the activity of the brain.
While fluorescent materials emit their own light, they still depend on some other light shining on them as a trigger. The next level down is bioluminescence, where light and colours are emitted by biological processes happening within the body of an organism.
Fireflies don’t need a light shining on them to glow: it’s all managed through chemical reactions. And while fireflies are literally just a light, bioluminescence is also used by marine animals such as the firefly squid—known in Japan as the hotaru ika — whose lights are scattered across their bodies, creating fantastic patterns on the surface of the water.
Bioluminescence is common in marine animals such as jellyfish and fish for signalling each other, especially where the amount of light that reaches through the water may not be sufficient for fluorescence.
If you’ve been following along so far, you might’ve noticed a pattern here. First, we have pigmentation, which depends on the light that shines on it. Then there’s fluorescence, which has its own colour but needs some light — any light — as a trigger. And finally, there’s bioluminescence which creates pre-set colours based on chemical reactions themselves.
While each of these technologies is more advanced than the last, there’s also a downside: resources. Pigments are something that a mere rock can manage, but bioluminescence needs a whole biological and chemical apparatus to power it.
So what happens if there aren’t enough resources for such advanced forms of colour? The fourth and final technique, which researchers have only recently been looking into, is structural colour. Rather than going along the route of “make your own light”, this technique takes us back to basics; back to Newton’s prism itself.
If you take apart a fallen peacock feather and chop it into fine pieces, hoping to reuse the colour for a dye or a paint, you’ll be in for a disappointment. The moment it gets broken apart, the peacock feather will lose all its colour, because the colour depends on the structure of the feather itself.
Imagine a very small version of Newton’s prism that splits light into a very small rainbow. Now, modify the shape with other bits and pieces of glass and mirrors, so that a few colours at the beginning and end are cut off. Actually, fine-tune it further so that most of the colours are cut off, leaving just one in the middle: the one we want. Unlike pigmentation, which absorbs all the unwanted colours and discards them, this tiny prism bends the light and converts it into the correct one.
Now imagine an entire organised array of such prisms — prisms so small, mind you, that thousands of them fit on a peacock’s feather or on a butterfly’s wing. They could be spaced periodically so that the light reflected from each individual structure interferes to produce new wavelengths.
That is the intricate beauty of structural colour.
Once you understand structural colour, it’s easy to see its advantages. To start with, structural colour is always brighter than pigments, because there’s no light energy absorbed in the process: whatever goes in comes back out.
The other advantage is that structural colours don’t fade away over time. Pigments fade, or become less effective; bioluminescent creatures eventually die. But structural colours is just a matter of having tiny glass-like structures in the same place, so they don’t fade away any more than a mirror does.
Scientists have of course been making the most of this idea. Pure Structural Colour is an emerging technology that uses nanoparticles, arranged in just the right way, to create the “boldest, brightest colour on Earth”. On display at the Royal Botanical Gardens, Kew, are round disks of Pure Structural Colour imitating the shades of butterfly wings. Some artists photograph these coloured disks in natural spots, giving the area a surreal “somebody copy-pasted a circle onto the photo” look.
Given the technology, none of this is very surprising. What is surprising, though, is that, with luck, people can soon start generating structural colours on their home printer.
In September 2021, a group of researchers used glass instead of paper to test out their printing, and they replaced pigments in the cartridges with a transparent polymer. This ‘clear ink’ was sprayed onto the glass, drop by drop, where it collected in little domes — think window raindrops, but much tinier in size.
When light interacts with these domes, they act like our mini-prisms: producing different colours, which you can vary by adjusting the size and shape of the drop. And it went beyond generic tweaking; they could control separate properties of the colours like hue, saturation, and brightness. Since the domes were printed only on one side, the colours were visible only on that side: the other side appeared transparent.
If it works out, this new structural-colour printer could be cheaper than usual, as it involves a single ink instead of four. Unlike pigments, which fade over time, the colours of these microdome structures would appear as fresh as they were first printed.
So far, the printer was developed for glass materials and might be used to create artistic designs for glass windows and billboards. It isn’t yet suitable for paper documents, though, so we might have to wait a while before we start seeing them at home.
But until then, we still have butterflies.